Hello Interactors,
Summer is waning and nature’s energy is draining. Meanwhile, record heat and fires in the northern hemisphere remind us the sun has yet to relent. But plants know what to do to prepare.
They slow down and repair. They store and restore through the winter snore for when the sun comes back for more. Why can’t we humans, and our societies, learn this rhythm of life? Maybe we’re just not as smart as plants. Yet.
Hey, Interplace hit 1,000 subscribers! 🙌🏼
Thanks, Interactors, for your energy and care. While I do this mostly for my own education, it’s nice to see people join the journey. Please give me a like and a hello so Interplace can continue to grow!
Now let’s go…
IS A FALL UPON US?
I can sense summer ending. And so can plants. They’re in the waning days of the growing season as they deftly adapt their metabolism – triggered by subtle shifts in the environment. As the sunlight fades and temperatures dip, the once vibrant act of photosynthesis slows – a signal from a rotating earth to take a pause. The production of glucose, their lifeblood, drops, but the tireless rhythm of respiration continues its steady beat.
Plants astutely sense the inevitable chill embarking on a strategic storage mission, hoarding precious energy and nutrients in their vaulted roots and stems. Stiffening to embrace the chilling challenge, they undergo a metamorphosis augmenting their resilience to freezing temperatures. Some species, like many of my sedums, channel their remaining vigor into blossoms and seeds before a winter's nap.
A plants' photochemical metabolic efficiency and prowess not only evokes wonder and admiration, but a bit of jealousy. Imagine being able to generate energy just by lying in the sun.
Metabolism is a biological process that determines how fast energy is exchanged between an organism and its surroundings. It transforms what's around us into fuel for maintenance, growth, and reproductive abundance.
This concept isn't just limited to individual organisms, but collections of organisms. Like groups of social humans. 'Sociometabolism' expands the idea of biological metabolism to human societies – how energy flows between a society and its environment, and how it's used within that society.
The sources of energy are different for each context. Biological metabolism relies mostly on light captured by plants which is then consumed by other living beings. A continuous metabolic flow through the entire food chain.
Sociometabolism can be more versatile. Sure, it involves consuming biomass for food or fuel, but it also encompasses energy generated from other sources – like fossil fuels, nuclear, solar, and wind power.
Both biological metabolism and a big chunk of sociometabolism depend heavily on consuming biological materials, whether it's plants or animals. This connects them closely to the flow of essential elements, like carbon, nitrogen, and phosphorus. Metabolism on earth is interconnected in a massive web of resource exchange.
Geographer Yadvinder Malhi wondered how much metabolic energy flows through the biosphere. He took a stab at estimating in a 2014 paper on “The Metabolism of a Human-Dominated Planet.”1 With the sun as the primary source of energy for the biosphere, he calculates about 174 petawatts (PW) of solar energy reaches the planet's upper atmosphere.
To put that in perspective, an old-fashioned 100-watt (W) lightbulb requires .1 kilowatts (KW) of energy. A 1000-watt drill requires 1 KW of energy. If one kilowatt is 103 W, then one petawatt is a whopping 1015 W. The sun is giving the planet 1 followed by 15 zeroes times 174 watts worth of energy! Plants and bacteria capable of photosynthesizing capture a portion of this solar energy through photosynthesis and store it as carbon-based chemical bonds.
This captured energy is quantified as Gross Primary Productivity (GPP), and the biosphere's GPP is approximately 210 petagrams of carbon per year. Converting GPP to energy units, the global photosynthetic metabolism is estimated at 265 terawatts (TW) or 1012 W. Sixty percent of that productivity comes from the land biosphere and 40% from the marine biosphere, representing only 0.2% of total surface solar energy.
Of that 0.2%, around 50-70% is used by plants and phytoplankton to metabolize and 30-50% is directed towards growing biomass. This biomass, in turn, is gobbled up by plant eaters, bacteria, and fungi. Fueling the ecosystem then requires about 75 TW of energy on land and 57 TW in the oceans to generate all the biomass energy available for other organisms to consume. Including humans. Given these numbers, how does our human metabolism stack up?
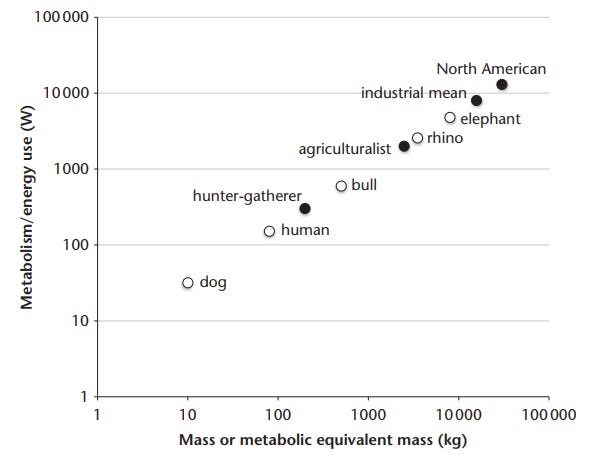
Imagine an 11x17 (A3) piece of paper sitting atop a leafy tree in a tropical forest in South America with the sun directly above. The amount of solar energy hitting that paper equals the energy required for an average sized active adult to metabolize one of our primary fuel sources – sugar. The equivalent of a 120 W light bulb. Cut the paper in half and that’s what would be required for an average-sized adult human to metabolize sugar while resting.
Any other mammal our size would require roughly the same amount of energy. However, we allocate energy differently than other mammals due to our relatively large brains. Nearly one-quarter to one-third of our energy, when resting, goes to feeding our brain. As a result, we dedicate proportionately less energy to growing biomass than a mammal of our size. In fact, we grow at a rate more akin to a reptile with similar mass. Malhi offers up a boa constrictor as a suitable comparison.
FROM HUNT AND FARM TO THE FAT FARM
That big brain of ours invents creative ways to consume energy relative to our biosphere family members. Especially when we humans congregate, socialize, and invent. Human sociometabolism has increased over our existence from hunting and gathering as other organisms do (preagriculture age), to controlling, appropriating, and domesticating plants and animals (agricultural age), to the use of biomass, fossil fuels, and other forms of energy to power inventions that feed our growing, and seemingly insatiable, sociometabolism (industrial age).
Even as increasingly more energy is now required to fuel servers to feed the current global wave of AI hysteria (information age), all three of these previous stages of sociometabolism still exist. It’s not so much that humans went from one stage to another, but more we added each stage to the other. Today, some communities and societies do more or less of hunting and gathering, farming, powering machines, or running software than others. Taken together, we are all part of human sociometabolism.
To quantify humanity's sociometabolism, Malhi considers both energy flows, which can be obtained from energy statistics in those societies that collect and publish them, and material flows, specifically the flow of biomass used for human or livestock nutrition. While energy flows are more easily accessible in contemporary contexts, material flows were a crucial aspect of the metabolism in pre-industrial societies and continue to hold significance in modern societies as well.
Malhi took some numbers from another study in 20082 that puts aspects of these sociometabolic transitions into perspective.
In a measure of population density per square kilometer, humans went from one human per 0.02–0.10 square kilometers in Preagricultural, to 40 in Agricultural, to 400 in Industrial.
Farmers as a percentage of the human population went from zero in Preagricultural, to greater than 80% in Agricultural, to less than 10% in Industrial.
Sociometabolism as watts per capita went from 300 W in Preagricultural, to 2000 W in Agricultural, to 8000 W in Industrial.
Total energy use per unit area (W per square kilometer) went from 6-30 W in Preagricultural, to 82,000 in Agricultural, to 3,300,000 in Industrial.
The share of plant and animal metabolism required to feed our own sociometabolism went from 0.0002% in Preagricultural, to 3-6% in Agricultural, to 210% in Industrial.
However, our big brains did invent ways to become more efficient with energy use. The percentage of biomass energy needed to fuel human sociometabolism went from greater than 99% in the Preagricultural age – that is, if you didn’t use your limited energy to hunt or gather – and/or socialize – you’d likely die, to greater than 95% in Agricultural – in other words, farming reduced the area needed to find food but still required a lot of energy, to 10-30% in Industrial – now those humans most advantaged by machines could use less of their own energy to live (and presumably store more energy in the form of fat).
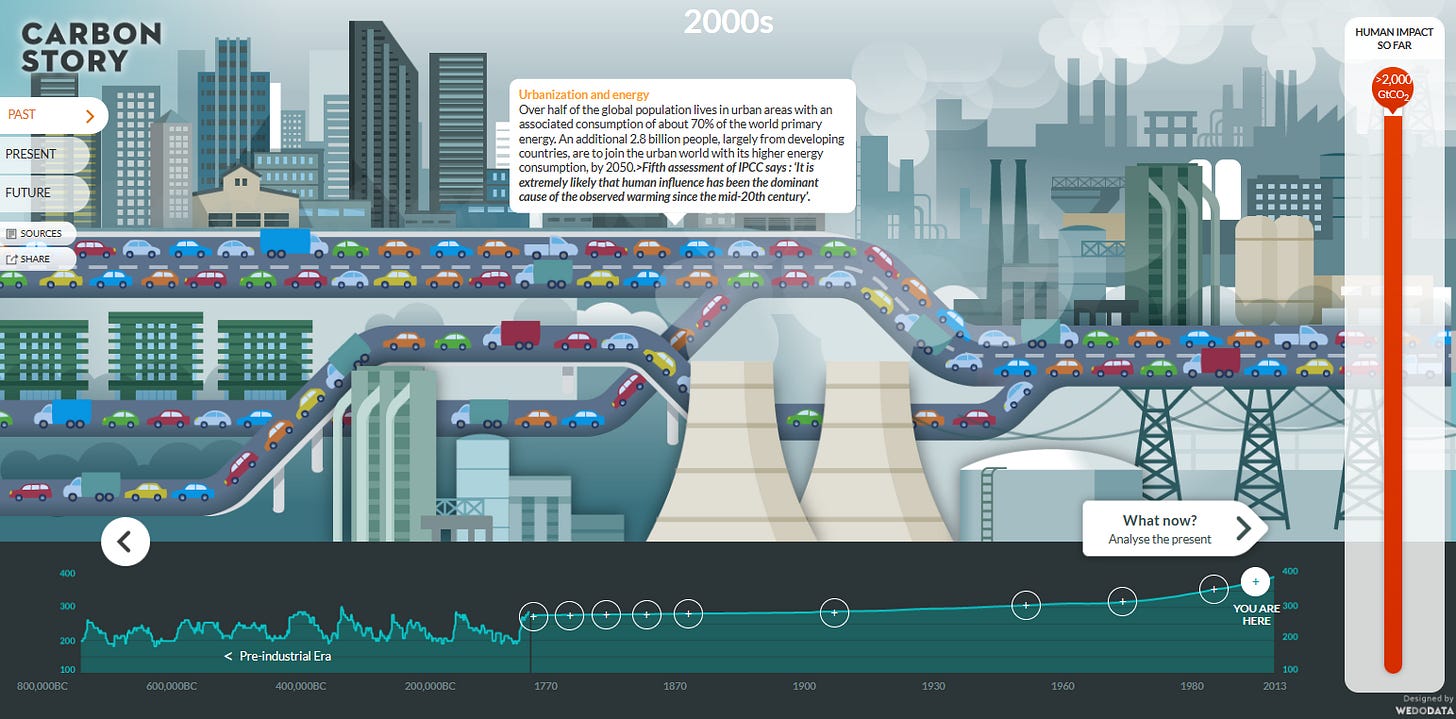
Human sociometabolism, for a vast and growing segment of the world’s population, has emerged as significantly smaller than the biological metabolism of the African savannah landscape where our species, Homo sapiens, first appeared. By Malhi’s calculations, our sociometabolism differs from theirs by six orders of magnitude.
For over a million years, Homo sapiens, and its ancestral Homo species, lived as hunter-gatherers. They improved little in resource gathering efficiency, despite gradual brain expansion and technological advancements. In this period, our metabolic impact on the planet remained relatively modest, likely lesser than that of much larger mammals like elephants and mammoths. However, a significant shift occurred about ten thousand years ago with the Neolithic revolution and the introduction of farming, marking a pivotal moment in human history.
The question is, is there enough energy on this planet to feed growing insatiable sociometabolisms? The trends don’t run in our favor unless human societies evolve as plants did. To survive, they cued into the natural rhythm of a rotating earth to form their own rhythm of life. Some ancient plants failed to ease their hyperactive, energy consuming process of photosynthesis. They didn’t take a break, conserve energy in their vaulted roots, repair damaged cells, and prepare for another trip around the sun. And evolution punished them. Now they’re fossilized carbon buried deep in the ground fueling our human sociometabolism.
Are we humans, as a species, future fossils in our waning days of a failed evolutionary experiment? Have we learned to slow our pace of consumption, conserve and store our energy? Can we evolve our sociometabolism by inventing rhythmic ways to seasonally repair and prepare ourselves and our societies for another growth spurt later in the journey around the sun? Are we, as a global society and species, adaptive thriving plants with an evolving future, or another example of failed future fossils?
The Metabolism of a Human-Dominated Planet. Yadvinder Malhi. 2014.
The Global Sociometabolic Transition. Journal of Industrial Ecology. Krausmann, F. et al. 2008.
Share this post